Articles & Issues
- Language
- korean
- Conflict of Interest
- In relation to this article, we declare that there is no conflict of interest.
- Publication history
-
Received April 1, 2014
Accepted May 14, 2014
-
This is an Open-Access article distributed under the terms of the Creative Commons Attribution Non-Commercial License (http://creativecommons.org/licenses/bync/3.0) which permits unrestricted non-commercial use, distribution, and reproduction in any medium, provided the original work is properly cited.
Copyright © KIChE. All rights reserved.
All issues
전산유체역학을 이용한 Fischer-Tropsch 마이크로채널 반응기의 채널 구조 영향 분석
Computational Fluid Dynamics Study of Channel Geometric Effect for Fischer-Tropsch Microchannel Reactor
서울대학교 화학생물공학부, 151-744 서울시 관악구 관악로 1
School of chemical and biological engineering, 1 Gwanak-ro, Gwanak-gu, Seoul 151-744, Korea
chhan@snu.ac.kr
Korean Chemical Engineering Research, December 2014, 52(6), 826-833(8), 10.9713/kcer.2014.52.6.826 Epub 1 December 2014
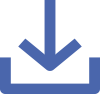
Abstract
해양 중소규모 가스전의 경제성에 대한 화두가 던져진 이후 전통 석유의 가격변동과 세계적인 환경규약 등에 맞물려 석유화학관련 산업계에서는 이를 효과적으로 대처하고 천연가스를 활용할 수 있는 공정을 개발하고자 하였다. 이에 Fischer-Tropsch 반응을 기반으로 하는 해상 GTL 공정(offshore gas-to-liquid process)이 제안되었고 부유시스템 platform으로 공정을 적용시키고자 마이크로채널 반응기가 떠오르고 있다. 본 논문에서는 단일 마이크로채널 반응기를 Fischer-Tropsch 반응을 기반으로 하여 Matlab과 ASPEN Hysys를 연동하여 모사하고 이로 얻어진 반응열을 도입해 상용 전산유체역학(computational fluid dynamics, CFD) 소프트웨어인 ANSYS fluent로 멀티 마이크로채널 반응기 모델을 제작하였다. 그리고 4가지의 설계변수인 냉각채널 넓이, 높이, 냉각채널과 반응채널의 간격, 냉각채널 간의 간격을 설정하고 이들의 변화에 따른 열유동을 3가지의 변수인 열유속, 냉각 및 반응채널의 최대온도의 변화를 시각화하여 그 경향성을 확인하였다. 경향성 분석 결과, 냉각채널의 넓이와 높이는 짧을수록 총 열유속이 높아졌으며 최대온도 역시 높아졌으나 냉각채널과 반응채널의 간격은 열유동에 거의 영향을 미치지 못하였다. 냉각채널 간의 간격은 짧을수록 총열유속이 높아졌으며 최대온도는 낮아졌다. 따라서 적절한 냉각채널의 넓이와 높이를 제안하고 짧은 간격의 냉각채널 구조를 도입하여 반응채널의 열량을 충분히 제거할 수 있는 반응기설계에 대한 휴리스틱을 제안할 수 있었다. 이처럼_x000D_
멀티채널 반응기의 모델을 설계하고 이로부터 적절한 변수를 선택해 그 경향성을 확인할 수 있는 방법을 통해 설계 단계에서부터 적절한 반응기 구조에 대한 제안을 하는데 도움을 줄 것이다.
Driven by both environmental and economic reasons, the development of small to medium scale GTL(gasto-liquid) process for offshore applications and for utilizing other stranded or associated gas has recently been studied increasingly. Microchannel GTL reactors have been prefrered over the conventional GTL reactors for such applications, due to its compactness, and additional advantages of small heat and mass transfer distance desired for high heat transfer performance and reactor conversion. In this work, multi-microchannel reactor was simulated by using commercial CFD code, ANSYS FLUENT, to study the geometric effect of the microchannels on the heat transfer phenomena. A heat generation curve was first calculated by modeling a Fischer-Tropsch reaction in a single-microchannel reactor model using Matlab-ASPEN integration platform. The calculated heat generation curve was implemented to the CFD model. Four design variables based on the microchannel geometry namely coolant channel width, coolant channel height, coolant channel to process channel distance, and coolant channel to coolant channel distance, were selected for calculating three dependent variables namely, heat flux, maximum temperature of coolant channel, and maximum temperature of process channel. The simulation results were visualized to understand the effects of the design variables on the dependent variables. Heat flux and maximum temperature of cooling channel and process channel were found to be increasing when coolant channel width and height were decreased. Coolant channel to process channel distance was found to have no effect on the heat transfer phenomena. Finally, total heat flux was found to be increasing and maximum coolant channel_x000D_
temperature to be decreasing when coolant channel to coolant channel distance was decreased. Using the qualitative trend revealed from the present study, an appropriate process channel and coolant channel geometry along with the distance between the adjacent channels can be recommended for a microchannel reactor that meet a desired reactor performance on heat transfer phenomena and hence reactor conversion of a Fischer-Tropsch microchannel reactor.
Keywords
References
Oxford Catalysts Group PLC., Technical experts’ report on the Velocys technology prepared by Nexant, Inc., 27 (2008)
Jun KW, KCS, 36(3), 8 (2009)
TOYO EGINEERING, Automation Systems, 8, 82 (2012)
Jarosch KT, Tonkovich ALY, Perry ST, Kuhlmann D, Wang Y, ACS Symp. Ser., 914, 258 (2005)
Bajus M, Petroleum & Coal, 54, 294 (2012)
Wang Y, Vanderwiel DP, Tonkovich ALY, Gao Y, Baker EG, “Catalyst Structure and Method of Fischer-tropsch Synthesis,” U.S. Patent No. 6, 451, 864 (2002)
Arzamendi G, Dieguez PM, Montes M, Odriozola JA, Sousa-Aguiar EF, Gandia LM, Chem. Eng. J., 160(3), 915 (2010)
Kandlikar SG, Upadhye HR, “Extending the Heat Flux Limit with Enhanced Microchannels in Direct Single-phase Cooling of Computer Chips,” Semiconductor Thermal Measurement and Management Symposium 2005 IEEE Twenty First Annual IEEE, 8-15 (2005)
An H, Li A, Sasmito AP, Kurnia JC, Jangam SV, Mujumdar AS, Chem. Eng. Sci., 75, 85 (2012)
Kusakabe K, Morooka S, Maeda H, Korean J. Chem. Eng., 18(3), 271 (2001)
Yang YI, Chun DH, Park JC, Jung H, Korean Chem. Eng. Res., 50(2), 358 (2012)
Guettel R, Turek T, Chem. Eng. Sci., 64(5), 955 (2009)
Derevich IV, Ermolaev VS, Mordkovich VZ, Galdina DD, Theor. Found. Chem. Eng., 46(1), 8 (2012)
Van der Laan GP, Beenackers AACM, Catal. Rev.-Sci. Eng., 41(3-4), 255 (1999)
Yates IC, Satterfield CN, Energy Fuels, 5(1), 168 (1991)
Atashi H, Siami F, Mirzaei AA, Sarkari M, J. Ind. Eng. Chem., 16(6), 952 (2010)
Fazlollahi F, Sarkari M, Zare A, Mirzaei AA, Mirzaei H, J. Ind. Eng. Chem., 18(4), 1223 (2012)
Krishna KR, Bell AT, J. Catal., 139(1), 104 (1993)
Knochen J, Guttel R, Knobloch C, Turek T, Chem. Eng. Process., 49(9), 958 (2010)
Post M, Van’t Hoog A, Minderhoud J, Sie S, AIChE J., 35, 1107 (1989)
Menter FR, AIAA J., 32, 1598 (1994)
Derevich IV, Ermolaev VS, Mordkovich VZ, Theor. Found. Chem. Eng., 42, 216 (2008)
Lee CJ, Lim Y, Kim HS, Han C, Ind. Eng. Chem. Res., 48, 794 (2008)
Derevich IV, Ermolaev VS, Zol’nikova NV, Mordkovich VZ, Theor. Found. Chem. Eng., 47, 191 (2013)
Jun KW, KCS, 36(3), 8 (2009)
TOYO EGINEERING, Automation Systems, 8, 82 (2012)
Jarosch KT, Tonkovich ALY, Perry ST, Kuhlmann D, Wang Y, ACS Symp. Ser., 914, 258 (2005)
Bajus M, Petroleum & Coal, 54, 294 (2012)
Wang Y, Vanderwiel DP, Tonkovich ALY, Gao Y, Baker EG, “Catalyst Structure and Method of Fischer-tropsch Synthesis,” U.S. Patent No. 6, 451, 864 (2002)
Arzamendi G, Dieguez PM, Montes M, Odriozola JA, Sousa-Aguiar EF, Gandia LM, Chem. Eng. J., 160(3), 915 (2010)
Kandlikar SG, Upadhye HR, “Extending the Heat Flux Limit with Enhanced Microchannels in Direct Single-phase Cooling of Computer Chips,” Semiconductor Thermal Measurement and Management Symposium 2005 IEEE Twenty First Annual IEEE, 8-15 (2005)
An H, Li A, Sasmito AP, Kurnia JC, Jangam SV, Mujumdar AS, Chem. Eng. Sci., 75, 85 (2012)
Kusakabe K, Morooka S, Maeda H, Korean J. Chem. Eng., 18(3), 271 (2001)
Yang YI, Chun DH, Park JC, Jung H, Korean Chem. Eng. Res., 50(2), 358 (2012)
Guettel R, Turek T, Chem. Eng. Sci., 64(5), 955 (2009)
Derevich IV, Ermolaev VS, Mordkovich VZ, Galdina DD, Theor. Found. Chem. Eng., 46(1), 8 (2012)
Van der Laan GP, Beenackers AACM, Catal. Rev.-Sci. Eng., 41(3-4), 255 (1999)
Yates IC, Satterfield CN, Energy Fuels, 5(1), 168 (1991)
Atashi H, Siami F, Mirzaei AA, Sarkari M, J. Ind. Eng. Chem., 16(6), 952 (2010)
Fazlollahi F, Sarkari M, Zare A, Mirzaei AA, Mirzaei H, J. Ind. Eng. Chem., 18(4), 1223 (2012)
Krishna KR, Bell AT, J. Catal., 139(1), 104 (1993)
Knochen J, Guttel R, Knobloch C, Turek T, Chem. Eng. Process., 49(9), 958 (2010)
Post M, Van’t Hoog A, Minderhoud J, Sie S, AIChE J., 35, 1107 (1989)
Menter FR, AIAA J., 32, 1598 (1994)
Derevich IV, Ermolaev VS, Mordkovich VZ, Theor. Found. Chem. Eng., 42, 216 (2008)
Lee CJ, Lim Y, Kim HS, Han C, Ind. Eng. Chem. Res., 48, 794 (2008)
Derevich IV, Ermolaev VS, Zol’nikova NV, Mordkovich VZ, Theor. Found. Chem. Eng., 47, 191 (2013)