Articles & Issues
- Language
- korean
- Conflict of Interest
- In relation to this article, we declare that there is no conflict of interest.
- Publication history
-
Received November 24, 2022
Revised January 6, 2023
Accepted January 13, 2023
-
This is an Open-Access article distributed under the terms of the Creative Commons Attribution Non-Commercial License (http://creativecommons.org/licenses/bync/3.0) which permits unrestricted non-commercial use, distribution, and reproduction in any medium, provided the original work is properly cited.
All issues
수소 및 탄소소재 생산을 위한 메탄 유동층 촉매분해 기술의 최근 동향
Recent Progress in the Catalytic Decomposition of Methane in a Fluidized Bed for Hydrogen and Carbon Material Production
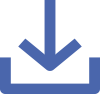
Abstract
화석연료를 대체할 수 있는 친환경 미래 에너지로 수소에너지에 대한 전세계적 관심이 높아지고 있다. 이에 따라 미
생물, 원자력 등을 이용한 차세대 수소 생산 기술이 개발되고 있으나, 화석연료 기반의 수소 생산 비용을 뛰어 넘기에는
아직 많은 시간과 노력이 필요한 상황이다. 화석연료 기반의 수소 생산 과정에서 온실가스의 배출량을 최소화 할 수
있는 방안으로 메탄 직접분해 반응 기술이 최근 관심을 모으고 있다. 공정의 경제성 향상을 위해서 수소 생산과 동시에
생산된 탄소물질의 고부가화 대한 연구가 필수적이며, 고부가 탄소 물질 중 하나인 탄소나노튜브(Carbon nanotube,
CNT)의 품질 및 수율 등과 관련한 촉매반응 연구가 지속되어 왔다. 또한 공정기술 측면에서, 연속적인 생산이 가능하
며 기체-고체 접촉 효율이 좋은 유동층 공정을 적용시켜 생산성과 경제성을 확보하고자 하는 연구가 시도되었다. 최근
유동층을 이용한 메탄 직접분해 반응기술은 수소 270 kg/day, 탄소 1000 kg/day의 생산이 가능한 정도의 기술 개발이
진행되었으며, 향후 촉매 재활성화, 분리 및 재순환 기술 등이 개발되면 공정의 효율이 크게 제고될 것이다. 이에 본
총설에서는 메탄 직접 분해에 활용되는 촉매 및 유동층 메탄 열분해 기술의 최근 연구들을 고찰하였다.
Global interest in hydrogen energy is increasing as an eco-friendly future energy that can replace fossil
fuels. Accordingly, a next-generation hydrogen production technology using microorganisms, nuclear power, etc. is
being developed, while a lot of time and effort are still required to overcome the cost of hydrogen production based on
fossil fuels. As a way to minimize greenhouse gas emissions in the hydrocarbon-based hydrogen production process,
methane direct decomposition technology has recently attracted attention. In order to improve the economic feasibility
of the process, the simultaneous production of value-added carbon materials with hydrogen can be one of the most
essential aspects. For that purpose, various studies on catalysis related to the quality and yield of high-value carbon
materials such as carbon nanotubes (CNTs). In terms of process technology, a number of the research and development
of fluidized-bed reactors capable of continuous production and improved gas-solid contact efficiency has been
attempted. Recently, methane direct decomposition technology using a fluidized bed has been developed to the extent that it can produce 270 kg/day of hydrogen and 1000 kg/day of carbon. Plus, with the development of catalyst
regeneration, separation and recirculation technologies, the process efficiency can be further improved. This review
paper investigates the recent development of catalysts and fluidized bed reactor for methane direct pyrolysis to identify
the key challenges and opportunities.
References
2. Castro, J., Fraile, D., Barth, F., Vanhoudt, W., Altmann, M. and Weindorf, W., “Technical Report on the Definition of ‘CertifHy Green’Hydrogen,” Brussels, Belgium 26 October, 2015(2015).
3. Dipu, A. L., “Methane Decomposition Into COx-free Hydrogen Over a Ni-based Catalyst: An Overview,” Int. J. Energy Res.,45(7), 9858-9877(2021).
4. Qian, J. X., Chen, T. W., Enakonda, L. R., Liu, D. B., Basset, J.M., and Zhou, L., “Methane Decomposition to Pure Hydrogen
and Carbon Nano Materials: State-of-the-art and Future Perspectives,” Int. J. Hydrog. Energy, 45(32), 15721-15743(2020). 5. Dufour, J., Gálvez, J. L., Serrano, D. P., Moreno, J. and Martínez,
G., “Life Cycle Assessment of Hydrogen Production by Methane Decomposition Using Carbonaceous Catalysts,” Int. J. Hydrog.Energy, 35(3), 1205-1212(2010).
6. Dufour, J., Serrano, D. P., Gálvez, J. L., González, A., Soria, E.,and Fierro, J. L., “Life Cycle Assessment of Alternatives for
Hydrogen Production from Renewable and Fossil Sources,” Int.J. Hydrog. Energy, 37(2), 1173-1183(2012).
7. Net Zero by 2050 – Analysis – IEA, “https://www.iea.org/reports/net-zero-by-2050,” (accessed November 16, 2022).
8. Keipi, T., Tolvanen, K. E., Tolvanen, H. and Konttinen, J., “ThermoCatalytic Decomposition of Methane: The Effect of Reaction Parameters on Process Design and the Utilization Possibilities of the Produced Carbon,” Energy Convers. Manag., 126, 923-934(2016).
9. Keipi, T., Hankalin, V., Nummelin, J. and Raiko, R., “Technoeconomic Analysis of Four Concepts for Thermal Decomposition of Methane: eduction of CO2 Emissions in Natural Gas Combustion,” Energy Convers. Manag., 110, 1-12(2016).
10. Global CNT Materials Market Size, Manufacturers, Supply Chain,Sales Channel and Clients, 2022-2028 “https://www.marketresearch.com/QYResearch-Group-v3531/Global-CNT-Materials-Size-Manufacturers-32048577/,” (accessed January 2, 2023).
11. Daloz, W., Scheiff, F., Ehrhardt, K., Flick, D. and Bode, A.,“The Quest for CO2-free Hydrogen—methane Pyrolysis at Scale,”US DOE ARPA-E Methane Cohort Kickoff (2019).
12. Schneider, S., Bajohr, S., Graf, F. and Kolb, T., “State of the Art of Hydrogen Production via Pyrolysis of Natural Gas,” ChemBioEng Rev., 7(5), 150-158(2020).
13. Upham, D. C., Agarwal, V., Khechfe, A., Snodgrass, Z. R., Gordon,M. J., Metiu, H. and McFarland, E. W., “Catalytic Molten Metals for the Direct Conversion of Methane to Hydrogen and Separable Carbon,” Science., 358(6365), 917-921(2017).
14. Bode, A., Anderlohr, C., Bernnat, J., Flick, F., Glenk, F., Klingler, D.and Munera-Parra, A. “Solids and Fluid Products from Gas-FfPaG,Final Report,” BMBF FKZ 033RC1301 AG. Bundesministerium für Bildung und Forschung, Bonn (2018).
15. A. Abánades, T. G. Geißler, T. Wetzel. Patent WO2019/154732 A1(2019).
16. A. Abánades, T. G. Geißler, T. Wetzel. Patent EP3521241 A1 (2019).
17. Gaudernack, B. and Lynum, S., “Hydrogen from Natural Gas Without Release of CO2 to the Atmosphere,” Int. J. Hydrog. Energy,23(12), 1087-1093(1998).
18. Pohlenz, J. B. and Scott, N. H., “Method for Hydrogen Production by Catalytic Decomposition of a Gaseous Hydrocarbon Stream,”U.S. Patent No. 3,284,161(1966).
19. Muradov, N., “Thermocatalytic CO2-Free Production of Hydrogen and Carbon from Hydrocarbon Fuels,” Proceedings of the 2000 Hydrogen Program Review, May, California(2000).
20. Muradov, N., Chen, Z. and Smith, F., “Fossil Hydrogen with Reduced CO2 Emission: Modeling Thermocatalytic Decomposition of Methane in a Fluidized Bed of Carbon Particles,” Int. J.Hydrog. Energy, 30(10), 1149-1158(2005).
21. Cornejo, A., Lu, H. and Chua, H. T., “A Process of Controlling the Morphology of Graphite,” Patent AU-2016312962-B2(2019).
22. Cornejo, A. and Chua, H. T., “Process for Producing Hydrogen and Graphitic Carbon from Hydrocarbons,” U.S. Patent No.11,505,458(2022).
23. https://hazergroup.com.au/.
24. https://hazer.wpmudev.host/about/#hazerprocess.
25. Hwang, B., Ngo, S. I., Lim, Y. I., Seo, M. W., Park, S. J., Ryu,H. J. and Lee, D., “Reaction Characteristics of Ni-Based Catalyst Supported by Al2O3 in a Fluidized Bed for CO2 Methanation,”Catalysts, 12(11), 1346(2022).
26. Kim, S. W., “Effect of Height on CNT Aggregates Size and Shape in Freeboard Region of a Fluidized Bed,” Korean Chem.Eng. Res., 57(1), 105-110(2019).
27. Park, S., “Fluidization Characteristics in Fluidized Bed Reactors Operated in Subatmospheric Pressure,” Korean Chem. Eng. Res.,58(2), 307-312(2020).
28. Park, S. H. and Kim, S. W., “Characteristics of Heat Absorption by Gas in a Directly-irradiated Fluidized Bed Particle Receiver,”Korean Chem. Eng. Res., 59(2), 239-246(2021).
29. Go, E. S., Kang, S. Y., Seo, S. B., Kim, H. W. and Lee, S. H.,“Slug Characteristics in a Bubbling Fluidized Bed Reactor for Polymerization Reaction,” Korean Chem. Eng. Res., 58(4), 651-657(2020).
30. Dai, H., Rinzler, A. G., Nikolaev, P., Thess, A., Colbert, D. T.and Smalley, R. E., “Single-wall Nanotubes Produced by Metalcatalyzed Disproportionation of Carbon Monoxide,” Chem. Phys.Lett., 260(3-4), 471-475(1996)
31. Kumar, M. and Ando, Y., “Chemical Vapor Deposition of Carbon Nanotubes: a Review on Growth Mechanism and Mass Production,” J. Nanosci. Nanotechnol., 10(6), 3739-3758(2010).
32. Azam, M. A., Manaf, N. S. A., Talib, E. and Bistamam, M. S.A., “Aligned Carbon Nanotube from Catalytic Chemical Vapor Deposition Technique for Energy Storage Device: a Review,”Ionics., 19(11), 1455-1476(2013).
33. Schaper, A. K., Hou, H., Greiner, A. and Phillipp, F., “The Role of Iron Carbide in Multiwalled Carbon Nanotube Growth,” J.Catal., 222(1), 250-254(2004).
34. Lin, M., Tan, J. P. Y., Boothroyd, C., Loh, K. P., Tok, E. S. and Foo, Y. L., “Dynamical Observation of Bamboo-like Carbon Nanotube Growth,” Nano Lett., 7(8), 2234-2238(2007).
35. Yoshida, H., Takeda, S., Uchiyama, T., Kohno, H. and Homma,Y., “Atomic-scale in-situ Observation of Carbon Nanotube Growth from Solid State Iron Carbide Nanoparticles,” Nano Lett., 8(7),2082-2086(2008).
36. Hofmann, S., Sharma, R., Ducati, C., Du, G., Mattevi, C., Cepek,C. and Robertson, J., “In situ Observations of Catalyst Dynamics During Surface-bound Carbon Nanotube Nucleation,” Nano Lett., 7(3), 602-608(2007).
37. Srilatha, K., Bhagawan, D., Kumar, S. S., and Himabindu, V.,“Sustainable Fuel Production by Thermocatalytic Decomposition of Methane–A Review,” S Afr J. Chem. Eng., 24, 156-167(2017).
38. Urdiana, G., Valdez, R., Lastra, G., Valenzuela, M. Á. and Olivas,A., “Production of Hydrogen and Carbon Nanomaterials Using Transition Metal Catalysts Through Methane Decomposition,” Mater. Lett., 217, 9-12(2018).
39. Donphai, W., Phichairatanaphong, O., Klysubun, W. and Chareonpanich, M., “Hydrogen and Carbon Allotrope Production Through
Methane Cracking over Ni/bimodal Porous Silica Catalyst: Effect of Nickel Precursor,” Int. J. Hydrog. Energy, 43(48), 21798-21809 (201).
40. Meshkani, F., Rezaei, M. and Rastegarpanah, A., “Preparation and Improvement of Nickel Catalyst Supported Ordered Mesoporous Spherical Silica for Thermocatalytic Decomposition of Methane,” J. Energy Inst., 93(6), 2488-2496(2020).
41. Rategarpanah, A., Meshkani, F., Wang, Y., Arandiyan, H. and Rezaei, M., “Thermocatalytic Conversion of Methane to Highly Pure Hydrogen over Ni-Cu/MgO·Al2O3 Catalysts: Influence of Noble Metals (Pt and Pd) on the Catalytic Activity and Stability,” Energy Convers. Manag., 166, 268-280(2018).
42. Pudukudy, M., Yaakob, Z., Kadier, A., Takriff, M. S. and Hassan,N. S. M., “One-pot Sol-gel Synthesis of Ni/TiO2 Catalysts for Methane Decomposition Into COx Free Hydrogen and Multiwalled Carbon Nanotubes,” Int. J. Hydrog. Energy, 42(26), 16495-16513(2017).
43. Xu, M., Lopez-Ruiz, J. A., Kovarik, L., Bowden, M. E., Davidson,S. D., Weber, R. S., Wang, I. W., Hu, J. and Dagle, R. A., “Structure
Sensitivity and Its Effect on Methane Turnover and Carbon Coproduct Selectivity in Thermocatalytic Decomposition of Methane over Supported Ni Catalyst,” Appl. Catal. A: Gen., 611, 117967 (2021).
44. Gao, B., Wang, I. W., Ren, L., Haines, T. and Hu, J., “Catalytic Performance and Reproducibility of Ni/Al2O3 and Co/Al2O3 Mesoporous Aerogel Catalysts for Methane Decomposition,”Ind. Eng. Chem. Res., 58(2), 798-807(2018).
45. Manasa, K., Naresh, G., Kalpana, M., Sasikumar, B., Velisoju, V. K., Chary, K. V., Michalkiewicz, B. and Venugopal, A., “Improved
H2 Yields over Rice Husk Derived SiO2 Nanoparticles Supported Ni Catalyst During Non-oxidative Methane Cracking,” J.Energy Inst., 99, 73-81(2021).
46. Torres, D., Pinilla, J. L. and Suelves, I., “Screening of Ni-Cu Bimetallic Catalysts for Hydrogen and Carbon Nanofilaments Production via Catalytic Decomposition of Methane,” Appl. Catal.A: Gen., 559, 10-19(2018).
47. Esteves, L. M., Daas, A. A., Oliveira, H. A. and Passos, F. B.,“Influence of Space Velocity and Catalyst Pretreatment on COx Free Hydrogen and Carbon Nanotubes Production over CoMo/MgO Catalyst,” Int. J. Hydrog. Energy, 45(51), 27299-27311(2020).
48. Khan, U. M., Sarmad, Q., Anwar, M., Khoja, A. H., SA, M. A.,Khan, Z. S., Hassan, M. and Shakir, S., “Synthesis of Cobalt
Loaded Double Perovskite Sr2TiFeO6-δ (STF) as a Stable Catalyst for Enhanced Hydrogen Production via Methane Decomposition,” Int. J. Energy Res., 45(14), 20073-20088(2021).
49. Ko, D. H., Kang, S. C., Lee, C. W. and Im, J. S., “Effects of Support Porosity of Co-Mo/MgO Catalyst on Methane Catalytic Decomposition for Carbon and Hydrogen Production,” J. Ind.Eng. Chem., 112(25), 162-170(2022).
50. Kludpantanapan, T., Rattanaamonkulchai, R., Srifa, A., KooAmornpattana, W., Chaiwat, W., Sakdaronnarong, C., Charinpanitkul, T., Assabumrungrat, S., Wongsakulphasatch, S., AieamsamAung, P., Watanabe, R., Fukuhara, C. and Ratchahat, S., “Development of CoMo-X Catalysts for Production of H2 and CNTs from Biogas by Integrative Process,” J. Environ. Chem. Eng., 10(4),107901(2022).
51. Aieamsam-Aung, P., Nantapong, P., Rattanaamonkulchai, R.,Kludpantanapan, T., Srifa, A., Koo-Amornpattana, W., Sakdaronnarong, C., Suchamalawong, P., Reubroycharoen, P., Kiatphuengporn, S., Charinpanitkul, T., Assabumrungrat, S., Wongsakulphasatch,S., Eiad-ua, A., Watanabe, R., Fukuhara, C. and Ratchahat, S.,“Effect of CoMo Metal Loading on H2 and CNTs Production From Biogas by Integrative Process,” Int. J. Hydrog. Energy,47(98), 41444-41460(2022).
52. Esteves, L. M., Oliveira, H. A., Xing, Y. and Passos, F. B.,“Cobalt Supported on Carbon Nanotubes for Methane Chemical Vapor Deposition for the Production of New Carbon Nanotubes,”New J. Chem., 45(31), 14218-14226(2021).
53. Shah, M., Al Mesfer, M. K. and Danish, M., “Facile Synthesis of Co-Rh Bimetallic Catalysts for Methane Decomposition: Effect of Support Morphology,” Fuel, 330, 125596(2022).
54. Lobiak, E. V., Kuznetsova, V. R., Flahaut, E., Okotrub, A. V. and Bulusheva, L. G., “Effect of Co-Mo Catalyst Preparation and CH4/
H2 Flow on Carbon Nanotube Synthesis,” Fuller Nanotub. Car.N., 28(9), 707-715(2020).
55. Calgaro, C. O. and Perez-Lopez, O. W., “Graphene and Carbon Nanotubes by CH4 Decomposition over CoAl Catalysts,” Mater.
Chem. Phys., 226, 6-19(2019).
56. Henao, W., Cazaña, F., Tarifa, P., Romeo, E., Latorre, N., Sebastian, Delgado, J. J. and Monzón, A., “Selective Synthesis of Carbon
Nanotubes by Catalytic Decomposition of Methane Using CoCu/cellulose Derived Carbon Catalysts: A Comprehensive Kinetic Study,” Chem. Eng. J., 404, 126103(2021).
57. Park, S. J., Kim, K. D., Park, Y. S., Go, K. S., Kim, W., Kim, M., Nho, N. S. and Lee, D. H., “Effect of Reduction Conditions of Mo-Fe/MgO on the Formation of Carbon Nanotube in Catalytic Methane Decomposition,” J. Ind. Eng. Chem., 109, 384-396(2022).
58. Azab, M. A., Awadallah, A. E., Aboul-Enein, A. A. and Hassan, S. A., “Single-step Synthesis of Graphene Nanosheets-carbon Nanotubes Hybrid Structure by Chemical Vapor Deposition of Methane Using Fe-Mo-MgO Catalysts,” Fuller Nanotub. Car.N., 1-11(2022).
59. Wang, I. W., Kutteri, D. A., Gao, B., Tian, H. and Hu, J., “Methane Pyrolysis for Carbon Nanotubes and COx-free H2 over Transitionmetal Catalysts,” Energy Fuels, 33(1), 197-205(2018).
60. Alcázar, H. E., Chire, E., Vargas, M. M., Villagarcía, B. L., Neira,J., Contin, A. and Alcázar, L. O., “Production and Characterization of Carbon Nanotubes by Methane Decomposition over NiFe/Al2O3 Catalyst and Its Application as Nanofillers in Polypro-pylene Matrix,” Mater. Res. Express., 8(11), 115001(2021).
61. Yan, P., Zhang, K. and Peng, Y., “Study of Fe2O3-Al2O3 Catalyst Reduction Parameters and Conditions for Catalytic Methane Decomposition,” Chem. Eng. Sci., 250, 117410(2022).
62. El-Ahwany, O. M., Awadallah, A. E., Aboul-Enein, A. A., AbdelAzim, S. M., Aboul-Gheit, N. A. and Abo-EL-Enein, S. A., “Dual Growth of Graphene Nanoplatelets and Carbon Nanotubes Hybrid Structure via Chemical Vapor Deposition of Methane over FeMgO Catalysts,” Fuller Nanotub. Car. N., 28(6), 435-445(2020).
63. Tezel, E., Figen, H. E. and Baykara, S. Z., “Hydrogen Production by Methane Decomposition Using Bimetallic Ni-Fe Catalysts,”Int. J. Hydrog., 44(20), 9930-9940(2019).
64. Kutteri, D. A., Wang, I. W., Samanta, A., Li, L. and Hu, J., “Methane Decomposition to Tip and Base Grown Carbon Nanotubes
and COx-free H2 over Mono-and Bimetallic 3d Transition Metal Catalysts,” Catal. Sci. Technol., 8(3), 858-869(2018).
65. Gao, B., Wang, I. W., Ren, L. and Hu, J., “Catalytic Methane Decomposition over Bimetallic Transition Metals Supported on Composite Aerogel,” Energy Fuels, 33(9), 9099-9106(2019).
66. Shen, Y., Ge, M. and Lua, A. C., “Deactivation of Bimetallic Nickel-copper Alloy Catalysts in Thermocatalytic Decomposition of Methane,” Catal. Sci. Technol., 8(15), 3853-3862(2018).
67. Al Mesfer, M. K., Danish, M. and Shah, M., “Synthesis and Optimization of Hydrotalcite Derived Ni-Fe-Cu Based Catalysts for Catalytic Methane Decomposition Process Using the Design of Experiment Approach,” J. Taiwan Inst. Chem. Eng., 128, 370-379(2021).
68. Wang, P., Zhu, H., Huang, M., Wan, C., Li, D. and Jiang, L.,“Catalytic Methane Decomposition to Hydrogen and Carbon over Hydrotalcite-derivative Composition-uniform and Sintering-resistant Ni-Fe/Al2O3 Alloy Catalysts,” Int. J. Energy Res., 46(12),16810-16822(2022).
69. Torres, D., Pinilla, J. L. and Suelves, I., “Cobalt Doping of α-Fe/Al2O3 Catalysts for the Production of Hydrogen and High-quality Carbon Nanotubes by Thermal Decomposition of Methane,” Int.J. Hydrog., 45(38), 19313-19323(2020).
70. Ly, H. V., Tran, Q. K., Chun, B. H., Oh, C., Kim, J. and Kim, S.S., “Bio-oil Production from Fast Pyrolysis of Furniture Processing Residue,” Korean J. Chem. Eng., 38(2), 306-315(2021).
71. Qureshi, K. M., Lup, A. N. K., Khan, S., Abnisa, F. and Daud,W. M. A. W., “Effect of Temperature and Feed Rate on Pyrolysis oil Produced via Helical Screw Fluidized Bed Reactor,” Korean J. Chem. Eng., 38(9), 1797-1809(2021).
72. Lee, J. R., Kim, Y. H. and Won, Y. S., “Solid-state Reaction Between MoS2 and MoO3 in a Fluidized Bed Reactor,” Korean J. Chem. Eng., 38(9), 1791-1796(2021).
73. Tan, W., Du, S., He, Y., Lv, G., Ma, W., Xing, A. and Huang, J.,“Effects of Opening Design of Gas Distribution Plate on Fluidization of the Synthesis Process of Organosilicon Monomer,”Korean J. Chem. Eng., 39(8), 2034-2043(2022).
74. Sahu, A. K., Raghavan, V. and Prasad, B., “Influence of Frictional Packing Limit on Hydrodynamics and Performance of Gas-solid
Fluidized Beds,” Korean J. Chem. Eng., 37(12), 2368-2383(2020).
75. Jeong, S. W., Lee, J. H., Kim, J. and Lee, D. H., “Fluidization Behaviors of Different Types of Multi-walled Carbon Nanotubes
in Gas-solid Fluidized Beds,” J. Ind. Eng. Chem., 35, 217-223(2016).
76. Kim, S. W., “Effect of Particle Size on Carbon Nanotube Aggregates Behavior in Dilute Phase of a Fluidized Bed,” Processes,6(8), 121(2018).
77. Chu, D., Dong, Q., Bai, W. and He, Y., “Hydrodynamics Characteristics of Agglomerated Carbon Nanotube in a Tapered Fluidized Bed,” Part. Sci. Technol., 1-11(2022).
78. Bai, W., Chu, D. and He, Y., “Bubble Characteristic of Carbon Nanotubes Growth Process in a Tapered Fluidized Bed Reactor Without a Distributor,” Chem. Eng. J., 407, 126792(2021).
79. Kim, S. W., “Measurement of Carbon Nanotube Agglomerates Size and Shape in Dilute Phase of a Fluidized Bed,” Korean Chem.Eng. Res., 55(5), 646-651(2017).
80. Geldart, D., “Types of Gas Fluidization,” Powder Technol., 7(5),285-292(1973).
81. Peng, J., Sun, W., Han, H., Xie, L. and Xiao, Y., “Experimental and Numerical Simulation Study on the Hydrodynamic Characteristics of Spherical and Irregular-shaped Particles in a 3D Liquid-fluidized Bed,” Korean J. Chem. Eng., 39(11), 3165-3176(2022).
82. An, Z., Wang, H. and Zhang, Y., “Prediction of Defluidization Behavior Using Particle Apparent Viscosity,” Korean J. Chem.Eng., 39(10), 2875-2882(2022).
83. Lee, G. H., Follett, W., Park, K., Kim, D., Lee, J. and Halloran,S., “Biot Number Calibration of an Oxy-PFBC Combustor Through Computational Particle Fluid Dynamic Analysis,” Korean J. Chem.Eng., 39(4), 1086-1095(2022).
84. Wang, X. S., Rahman, F. and Rhodes, M. J., “Nanoparticle Fluidization and Geldart’s Classification,” Chem. Eng. Sci., 62(13),
3455-3461(2007).
85. Morooka, S., Kusakabe, K., Kobata, A. and Kato, Y., “Fluidization State of Ultrafine Powders,” J. Chem. Eng. Japan, 21(1), 41-
46(1988).
86. Valverde, J. M. and Castellanos, A., “Types of Gas Fluidization of Cohesive Granular Materials,” Phys. Rev. E, 75(3), 031306(2007).
87. Van Ommen, J. R., Valverde, J. M. and Pfeffer, R., “Fluidization of Nanopowders: a Review,” J. Nanopart Res., 14(3), 1-29(2012).
88. Yu, H., Zhang, Q., Gu, G., Wang, Y., Luo, G. and Wei, F., “Hydrodynamics and Gas Mixing in a Carbon Nanotube Agglomerate
Fluidized Bed,” AIChE J., 52(12), 4110-4123(2006).
89. Jeong, S. W. and Lee, D. H., “Estimation of Agglomerate Size of Multi-walled Carbon Nanotubes in Fluidized Beds,” Adv. Powder
Technol., 28(10), 2706-2712(2017).
90. Parmar, K. R., Pant, K. K. and Roy, S., “Blue Hydrogen and Carbon Nanotube Production via Direct Catalytic Decomposition of Methane in Fluidized Bed Reactor: Capture and Extraction of Carbon in the form of CNTs,” Energy Convers. Manag., 232,113893(2021).
91. Geng, S., Han, Z., Hu, Y., Cui, Y., Yue, J., Yu, J. and Xu, G.,“Methane Decomposition Kinetics over Fe2O3 Catalyst in Micro Fluidized Bed Reaction Analyzer,” Ind. Eng. Chem. Res., 57(25),8413-8423(2018).
92. Łamacz, A. and Łabojko, G., “CNT and H2 Production During CH4 Decomposition over Ni/CeZrO2. II. Catalyst Performance
and Its Regeneration in a Fluidized Bed,” ChemEngineering, 3(1),25(2019).
93. Bai, W., Yao, C., Chu, D., Geng, L. and He, Y., “Research on MWCNT Growth Process Through on-line Intermittent Monitoring in a Fluidized Bed Reactor,” Results Mater., 6, 100055(2020).
94. Qian, J. X., Enakonda, L. R., Wang, W. J., Gary, D., Del P., Basset, J. M., Liu, D. B. and Zhou, L., “Optimization of a Fluidized Bed Reactor for Methane Decomposition over Fe/Al2O3 Catalysts: Activity and Regeneration Studies,” Int. J. Hydrog.Energy, 44(60), 31700-31711(2019).
95. Keller, M., Matsumura, A. and Sharma, A., “Spray-dried Fe/Al2O3 as a Carbon Carrier for COx-free Hydrogen Production via Methane Cracking in a Fluidized Bed Process,” Chem. Eng. J.,398, 125612(2020).
96. Zhou, L., Enakonda, L. R., Li, S., Gary, D., Del-Gallo, P., Mennemann,C. and Basset, J. M., “Iron Ore Catalysts for Methane Decomposition to Make COx Free Hydrogen and Carbon Nano Material,” J. Taiwan Inst. Chem. Eng., 87, 54-63(2018).
97. Abánades, A., Rubbia, C. and Salmieri, D., “Thermal Cracking of Methane into Hydrogen for a CO2-free Utilization of Natural Gas,” Int. J. Hydrog. Energy, 38(20), 8491-8496(2013).
98. Wei, F., Wang, Y., Luo, G., Yu, H., Li, Z., Qian, W., Wang, Z.and Jin, Y., “Continuous Mass Production of Carbon Nanotubes in a Nano-agglomerate Fluidized-bed and the Reactor,” U.S. Patent No. 7,563,427(2009).
99. Denton, R. D., Noyes, D. B., Koveal Jr, R. J. and Ring, T. A.,“Removing Carbon Nanotubes from a Continuous Reactor Effluent,” U.S. Patent No. 10,343,104(2019).