Overall
- Language
- korean
- Conflict of Interest
- In relation to this article, we declare that there is no conflict of interest.
- Publication history
-
Received September 3, 2024
Revised September 18, 2024
Accepted September 20, 2024
-
This is an Open-Access article distributed under the terms of the Creative Commons Attribution Non-Commercial License (http://creativecommons.org/licenses/bync/3.0) which permits unrestricted non-commercial use, distribution, and reproduction in any medium, provided the original work is properly cited.
Most Cited
고효율 이산화탄소 메탄화 반응을 위한 저온 공정용 니켈 촉매 구조 및 시스템 연구 동향
Research Trends on the Structure and System of Nickel Catalysts for Low-Temperature Processes in Highly Efficient Carbon Dioxide Methanation Reactions
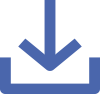
Abstract
본 총설은 저온 CO2 메탄화 반응을 위한 니켈 촉매의 최신 연구 동향을 종합적으로 검토하고, 특히 저온 CO2 메탄
화 반응을 위한 촉매 합성 및 반응기 설계에 중점을 둔다. 기존에 보고되었던 촉매 합성법인 함침법 이외에 최근 주목
받는 용매 열 합성법, 전기방사법으로 합성한 촉매의 구조적, 화학적 특성이 CO2 메탄화 반응에 미치는 영향을 분석
한다. 또한 반응 효율을 향상시키고자 3D 촉매 구조 및 촉매 적층형 반응기 설계를 기반으로 공정 비용 절감 및 스케
일업 가능성에 대해서 논의한다. 혁신적인 촉매 합성법을 통해 촉매의 산소 공공 및 결정 구조를 제어하고, 이에 촉매
의 3D 구조 및 반응기 설계를 통해 니켈의 활성 면적을 확보함으로써 CO2 메탄화 반응의 효율을 극대화할 수 있음을
규명한다. 본 총설이 주요 온실가스인 CO2를 감축함과 동시에 천연 가스를 대체할 수 있는 CH4로 전환하는 분야의 기
초자료로 활용될 것으로 기대된다.
This review comprehensively reviews the latest research trends in nickel catalysts for low-temperature CO2
methanation reactions, with special emphasis on catalyst synthesis and reactor design for low-temperature CO2 methanation
reactions. In addition to the previously reported catalyst synthesis method of impregnation, the effects of structural and
chemical properties of catalysts synthesized by solvothermal synthesis and electrospinning on CO2 methanation reactions
were analyzed. We also discuss the potential for process cost reduction and scale-up based on 3D catalyst structures and
catalyst stacked reactor designs to improve reaction efficiency. It is found that the efficiency of the CO2 methanation
reaction can be maximized by controlling the oxygen vacancies and crystal structure of the catalyst through an
innovative catalyst synthesis method, and by increasing the active area of nickel through the 3D structure of the catalyst
and the reactor design. It is expected that this review will serve as the basis for the field of converting natural gas into
CH4, which can be used as a substitute for natural gas while reducing CO2, a major greenhouse gas.
References
and Models,” Nature Climate Change, 3(1), 52-58(2013).
2. Coumou, D. and Rahmstorf, S., “A Decade of Weather Extremes,”
Nature Climate Change, 2(7), 491-496(2012).
3. Kossin, J. P., Knapp, K. R., Olander, T. L. and Velden, C. S.,
“Global Increase in Major Tropical Cyclone Exceedance Probability
over the Past Four Decades,” Proceedings of the National
Academy of Sciences, 117(22), 11975-11980(2020).
4. Van Oldenborgh, G. J., Otto, F. E., Haustein, K. and Cullen H.
“Climate Change Increases the Probability of Heavy Rains Like
Those of Storm Desmond in the UK–an Event Attribution Study
in Near-real Time,” Hydrology and Earth System Sciences Discussions,
12(12), 13197-13216(2015).
5. Odeyomi, O. A., Ejimakor, G. and Isikhuemhen, O. S., “Effects
of Climate Change on Crop Yield: Is it a Benefit or Menace?,”
ESI Preprints, 32, 42-42(2024).
6. Walsh, K. J., McBride, J. L., Klotzbach, P. J., Balachandran, S.,
Camargo, S. J., Holland, G., Knutson, T. R., Kossin, J. P., Lee, T.
C. and Sobel A., “Tropical Cyclones and Climate Change,” Wiley
Interdisciplinary Reviews: Climate Change, 7(1), 65-89(2016).
7. Qu, Q., Jian, S., Chen, A. and Xiao, C., “Investigating the Dynamic
Change and Driving Force of Vegetation Carbon Sink in Taihang
Mountain, China,” Land, 13(9), 1348(2024).
8. Ye, J., Fanyang, Y., Wang, J., Meng, S. and Tang, D., “A Literature
Review of Green Building Policies: Perspectives from Bibliometric
Analysis,” Buildings, 14(9), 2607(2024).
9. Yeh, C. H. and Chen, W. M., “Optimizing LCD Structures to
Mitigate Carbon Emissions Based on Root-mean-square Values,”
Applied Optics, 63(25), 6603-6615(2024).
10. Hasan, G. G., Laouini, S. E., Osman, A. I., Bouafia, A., Althamthami
M., Meneceur S., Al-Hazeef M. S., Al-Fatesh A. S. and Rooney,
D. W., “Green Synthesis of Mn3O4@ CoO Nanocomposites
Using Rosmarinus officinalis L. Extract for Enhanced Photocatalytic
Hydrogen Production and CO2 Conversion,” Journal of
Environmental Chemical Engineering, 113911(2024).
11. Ming, L., Dae-Yeong, K., Shutaro, N. and Tomohiro, N., “CO2
Methanation Through Gliding Arc Discharge Over Ni/Al2O3,”
International Journal of Plasma Environmental Science and Technology,
18(02), e02005-e02005(2024).
12. Farshchi, M. E., Asgharizadeh, K., Jalili, H., Nejatbakhsh, S.,
Azimi, B., Aghdasinia, H. and Mohammadpourfard, M., “Bimetallic
MOF@ CdS Nanorod Composite for Highly Efficient Piezophotocatalytic
CO2 Methanation under Visible Light,” Journal of
Environmental Chemical Engineering, 113909(2024).
13. Yang, C., Zhang, J., Liu, W., Cheng, Y., Yang, X. and Wang, W.,
“Rational H2 Partial Pressure over Nickel/Ceria Crystal Enables
Efficient and Durable Wide Temperature Zone Air Level CO2 Methanation,”
Chemistry–A European Journal, e202402516(2024).
14. Guo, L., Zhang, T., Qiu, J., Bai J., Li, Z., Wang H., Cai, X., Yang,
Y. and Xu, Y., “Cobalt-Doped Ni-Based Catalysts for Low-Temperature
CO2 Methanation,” Available at SSRN 4915073(2024).
15. Chopendra, G. W., Nakamura, M., Shimada, T., Machida, H. and
Norinaga, K., “6-2-3 Development of CO2 Methanation Catalysts
Supported on CeO2 And SiC At Elevated Temperatures,” Proceedings
of the Annual Conference of The Japan Institute of Energy,
The 33rd Annual Conference of the Japan Institute of Energy(2024).
16. Busca, G., Spennati, E., Riani, P. and Garbarino, G., “Looking
for An Optimal Composition of Nickel-based Catalysts for CO2
Methanation,” Energies, 16(14), 5304(2023).
17. Zainul, R., Ali, A. B., Jasim, D. J., Al-Bayati, A. D. J., Kaur, I.,
Kumar, A., Mahariq, I., Hasan, M. A., Islam, S. and Kareem, M.,
“Biphenyl Monolayer Construction with Single Transition Metal
Doping as Electrocatalysts for Conversion CO2 to Fuel,” International
Journal of Hydrogen Energy, (2024).
18. Anzola-Rojas, M. D. P., Eng, F., Fuess, L. T., Pozzi, E., Nolasco,
M. A., De Wever, H., Pant, D. and Zaiat, M., “Hydrogen Production
from Fermented Sugarcane Vinasse and its Utilization
by Biosynthesis Processes in a Single-Chambered Microbial Electrolysis
Cell, ” Available at SSRN 4914019.
19. Lin, L., Wang, K., Yang, K., Chen, X., Fu, X. and Dai, W., “The
Visible-light-assisted Thermocatalytic Methanation of CO2 over
Ru/TiO(2-x) Nx,” Applied Catalysis B: Environmental, 204, 440-
455(2017).
20. Do, J. Y., Park, N.-K., Seo, M. W., Lee, D., Ryu, H.-J. and Kang,
M., “Effective Thermocatalytic Carbon Dioxide Methanation on Ca-inserted NiTiO3 Perovskite,” Fuel, 271, 117624(2020).
21. Rusdan, N. A., Timmiati, S. N., Isahak, W. N. R. W., Yaakob, Z., Lim,
K. L. and Khaidar, D. “Recent Application of Core-shell Nanostructured
Catalysts for CO2 Thermocatalytic Conversion Processes,”
Nanomaterials, 12(21), 3877(2022).
22. Gao, J., Shiong, S. C. S. and Liu, Y., “Reduction of CO2 to
Chemicals and Fuels: Thermocatalysis Versus Electrocatalysis,”
Chemical Engineering Journal, 145033(2023).
23. Ashok, J., Pati, S., Hongmanorom, P., Tianxi, Z., Junmei, C. and
Kawi, S., “A Review of Recent Catalyst Advances in CO2 Methanation
Processes,” Catalysis Today, 356, 471-489(2020).
24. Simakov, D. S. and Simakov, D. S., “Thermocatalytic Conversion
of CO2,” Renewable Synthetic Fuels and Chemicals From
Carbon Dioxide: Fundamentals, Catalysis, Design Considerations
and Technological Challenges, 1-25(2017).
25. Lee, W. J., Li, C., Prajitno, H., Yoo, J., Patel, J., Yang, Y. and Lim,
S., “Recent Trend in Thermal Catalytic Low Temperature CO2
Methanation: A Critical Review,” Catalysis Today, 368, 2-19(2021).
26. Li, L., Zeng, W., Song, M., Wu, X., Li, G. and Hu, C., “Research
Progress and Reaction Mechanism of CO2 Methanation over Nibased
Catalysts at Low Temperature: A Review,” Catalysts, 12(2),
244(2022).
27. Wang, Y., Xu, Y., Liu, Q., Sun, J., Ji, S. and Wang, Z. J., “Enhanced
Low-temperature Activity for CO2 Methanation over NiMgAl/SiC
Composite Catalysts,” Journal of Chemical Technology & Biotechnology,
94(12), 3780-3786(2019).
28. Panagiotopoulou, P., “Hydrogenation of CO2 over Supported Noble
Metal Catalysts,” Applied Catalysis A: General, 542, 63-70 (2017).
29. Ocampo, F., Louis, B., Kiwi-Minsker, L. and Roger, A.-C., “Effect
of Ce/Zr Composition and Noble Metal Promotion on Nickel
Based CexZr1−xO2 Catalysts for Carbon Dioxide Methanation,”
Applied Catalysis A: General, 392(1-2), 36-44(2011).
30. Cui, Y., He, S., Yang, J., Gao, R., Hu, K., Chen, X., Xu, L., Deng,
C., Lin, C. and Peng, S., “Research Progress of Non-Noble Metal
Catalysts for Carbon Dioxide Methanation,” Molecules, 29(2),
374(2024).
31. Gac, W., Zawadzki, W., Rotko, M., Greluk, M., Słowik, G. and
Kolb, G. “Effects of Support Composition on the Performance of
Nickel Catalysts in CO2 Methanation Reaction,” Catalysis Today,
357, 468-482(2020).
32. Delmelle, R., Duarte, R. B., Franken, T., Burnat, D., Holzer, L.,
Borgschulte, A. and Heel, A., “Development of Improved Nickel
Catalysts for Sorption Enhanced CO2 Methanation,” International
Journal of Hydrogen Energy, 41(44), 20185-20191(2016).
33. Le, T. A., Kim, M. S., Lee, S. H., Kim, T. W. and Park, E. D., “CO
and CO2 Methanation over Supported Ni Catalysts,” Catalysis
Today, 293, 89-96(2017).
34. Liang, C., Zhang, L., Zheng, Y., Zhang, S., Liu, Q., Gao, G., Dong,
D., Wang, Y., Xu, L. and Hu, X. “Methanation of CO2 over Nickel
Catalysts: Impacts of Acidic/basic Sites on Formation of the Reaction
Intermediates,” Fuel, 262, 116521(2020).
35. Tsiotsias, A. I., Charisiou, N. D., Italiano, C., Ferrante, G. D., Pino,
L., Vita, A., Sebastian, V., Hinder, S. J., Baker, M. A. and Sharan,
A., “Ni-noble Metal Bimetallic Catalysts for Improved Low
Temperature CO2 Methanation,” Applied Surface Science, 646,
158945(2024).
36. Musab Ahmed, S., Ren, J., Ullah, I., Lou, H., Xu, N., Abbasi, Z.
and Wang, Z., “Ni Based Catalysts for CO2 Methanation: Exploring
the Support Role in Structure-Activity Relationships,” Chem-
SusChem, 17(9), e202400310(2024).
37. Baig, N., Kammakakam, I. and Falath, W., “Nanomaterials: A
Review of Synthesis Methods, Properties, Recent Progress, and
Challenges,” Materials Advances, 2(6), 1821-1871(2021).
38. Suo, G., Ahmed, S. M., Cheng, Y., Zhang, J., Li, Z., Hou, X., Yang,
Y., Ye, X., Feng, L. and Zhang, L., “Heterostructured CoS2/CuCo2S4@
N-doped Carbon Hollow Sphere for Potassium-ion Batteries,”
Journal of Colloid and Interface Science, 608, 275-283(2022).
39. Li, J. P. H., Zhou, X., Pang, Y., Zhu, L., Vovk, E. I., Cong, L.,
van Bavel, A. P., Li, S. and Yang, Y., “Understanding of Binding
Energy Calibration in XPS of Lanthanum Oxide by in situ Treatment,”
Physical Chemistry Chemical Physics, 21(40), 22351-
22358(2019).
40. Jin, B., Li, S. and Liang, X., “Enhanced Activity and Stability of
MgO-promoted Ni/Al2O3 Catalyst for Dry Reforming of Methane:
Role of MgO,” Fuel, 284, 119082(2021).
41. Zhang, M., Ye, J., Qu, Y., Lu, X., Luo, K., Dong, J., Lu, N., Niu,
Q., Zhang, P. and Dai, S., “Highly Stable and Selective Ni/ZrO2
Nanofiber Catalysts for Efficient CO2 Methanation,” ACS Applied
Materials & Interfaces, 16(27), 34936-34946(2024).
42. Hu, F., Ye, R., Jin, C., Liu, D., Chen, X., Li, C., Lim, K. H., Song,
G., Wang, T. and Feng, G., “Ni Nanoparticles Enclosed in Highly
Mesoporous Nanofibers with Oxygen Vacancies for Efficient
CO2 Methanation,” Applied Catalysis B: Environmental, 317,
121715(2022).
43. Vita, A., Italiano, C., Pino, L., Frontera, P., Ferraro, M. and Antonucci,
V., “Activity and Stability of Powder and Monolith-coated
Ni/GDC Catalysts for CO2 Methanation,” Applied Catalysis B:
Environmental, 226, 384-395(2018).
44. Frey, M., Edouard, D. and Roger, A.-C., “Optimization of Structured
Cellular Foam-based Catalysts for Low-temperature Carbon
Dioxide Methanation in a Platelet Milli-reactor,” Comptes Rendus.
Chimie, 18(3), 283-292(2015).
45. Ricca, A., Palma, V., Martino, M. and Meloni, E., “Innovative Catalyst
Design for Methane Steam Reforming Intensification,” Fuel,
198, 175-182(2017).
46. Götz, M., Lefebvre, J., Mörs, F., Koch, A. M., Graf, F., Bajohr,
S., Reimert, R. and Kolb, T., “Renewable Power-to-Gas: A technological
and Economic Review,” Renewable Energy, 85, 1371-
1390(2016).
47. Vita, A., Cristiano, G., Italiano, C., Specchia, S., Cipitì, F. and
Specchia, V., “Methane Oxy-steam Reforming Reaction: Performances
of Ru/γ-Al2O3 Catalysts Loaded on Structured Cordierite
Monoliths,” International Journal of Hydrogen Energy, 39(32),
18592-18603(2014).
48. Vita, A., Cristiano, G., Italiano, C., Pino, L. and Specchia, S., “Syngas
Production by Methane Oxy-steam Reforming on Me/CeO2
(Me=Rh, Pt, Ni) Catalyst Lined on Cordierite Monoliths,” Applied
Catalysis B: Environmental, 162, 551-563(2015).
49. Vita, A., Italiano, C., Fabiano, C., Lagana, M. and Pino, L., “Influence
of Ce-precursor and Fuel on Structure and Catalytic Activity
of Combustion Synthesized Ni/CeO2 Catalysts for Biogas Oxidative
Steam Reforming,” Materials Chemistry and Physics, 163,
337-347(2015).
50. Danaci, S., Protasova, L., Lefevere, J., Bedel, L., Guilet, R. and Marty, P., “Efficient CO2 Methanation over Ni/Al2O3 Coated
Structured Catalysts,” Catalysis Today, 273, 234-243(2016).
51. Junaedi, C., Hawley, K., Walsh, D., Roychoudhury, S., Abney,
M. and Perry, J., “Compact and Lightweight Sabatier Reactor
for Carbon Dioxide Reduction,” 41st International Conference
on Environmental Systems, (2011).
52. Lefevere, J., Gysen, M., Mullens, S., Meynen, V. and Van Noyen,
J., “The Benefit of Design of Support Architectures for Zeolite
Coated Structured Catalysts for Methanol-to-olefin Conversion,”
Catalysis Today, 216, 18-23(2013).
53. Ratchahat, S., Sudoh, M., Suzuki, Y., Kawasaki, W., Watanabe,
R. and Fukuhara, C., “Development of a Powerful CO2 Methanation
Process Using a Structured Ni/CeO2 Catalyst,” J. CO2 Util.
24, 210-219(2018).
54. Schlereth, D. and Hinrichsen, O., “A Fixed-bed Reactor Modeling
Study on the Methanation of CO2,” Chemical Engineering Research
and Design, 92(4), 702-712(2014).
55. Fukuhara, C., Hayakawa, K., Suzuki, Y., Kawasaki, W. and
Watanabe, R., “A Novel Nickel-based Structured Catalyst for CO2
Methanation: A Honeycomb-type Ni/CeO2 Catalyst to Transform
Greenhouse Gas Into Useful Resources,” Applied Catalysis A:
General, 532, 12-18(2017).
56. Schlereth, D., Donaubauer, P. J. and Hinrichsen, O., “Metallic
Honeycombs as Catalyst Supports for Methanation of Carbon
Dioxide,” Chemical Engineering & Technology, 38(10), 1845-1852
(2015).
57. Siakavelas, G. I., Charisiou, N. D., AlKhoori, S., AlKhoori, A. A.,
Sebastian, V., Hinder, S. J., Baker, M. A., Yentekakis, I., Polychronopoulou,
K. and Goula, M. A., “Highly Selective and Stable
Nickel Catalysts Supported on Ceria Promoted with Sm2O3, Pr2O3
and MgO for the CO2 Methanation Reaction,” Applied Catalysis
B: Environmental, 282, 119562(2021).
58. Alarcón, A., Guilera, J., Díaz, J. A. and Andreu, T., “Optimization
of Nickel and Ceria Catalyst Content for Synthetic Natural Gas
Production Through CO2 Methanation,” Fuel Processing Technology,
193, 114-122(2019).
59. Sholeha, N. A., Jannah, L., Rohma, H. N., Widiastuti, N., Prasetyoko,
D., Jalil, A. A. and Bahruji, H., “Synthesis of Zeolite NaY
From Dealuminated Metakaolin as Ni Support for CO2 Hydrogenation
to Methane,” Clays and Clay Minerals, 68(5), 513-523
(2020).
60. Everett, O. E., Zonetti, P. C., Alves, O. C., de Avillez, R. R. and
Appel, L. G. “The Role of Oxygen Vacancies in the CO2 Methanation
Employing Ni/ZrO2 doped with Ca,” International Journal
of Hydrogen Energy, 45(11), 6352-6359(2020).
61. Lin, J., Ma, C., Luo, J., Kong, X., Xu, Y., Ma, G., Wang, J., Zhang,
C., Li, Z. and Ding, M., “Preparation of Ni Based Mesoporous
Al2O3 Catalyst with Enhanced CO2 Methanation Performance,”
RSC Advances, 9(15), 8684-8694(2019).
62. Bukhari, S. N., Chong, C. C., Setiabudi, H. D., Cheng, Y. W.,
Teh, L. P. and Jalil, A. A., “Ni/Fibrous Type SBA-15: Highly Active
and Coke Resistant Catalyst for CO2 Methanation,” Chemical
Engineering Science, 229, 116141(2021).
63. Gnanakumar, E. S., Chandran, N., Kozhevnikov, I. V., Grau-Atienza,
A., Fernández, E. V. R., Sepulveda-Escribano, A. and Shiju, N.
R. “Highly Efficient Nickel-niobia Composite Catalysts for
Hydrogenation of CO2 to Methane,” Chemical Engineering Science,
194, 2-9(2019).
64. Jiang, Y., Huang, T., Dong, L., Su, T., Li, B., Luo, X., Xie, X.,
Qin, Z., Xu, C. and Ji, H., “Mn Modified Ni/bentonite for CO2
Methanation,” Catalysts, 8(12), 646(2018).
65. Aziz, M., Jalil, A., Triwahyono, S. and Sidik, S., “Methanation
of Carbon Dioxide on Metal-promoted Mesostructured Silica
Nanoparticles,” Applied Catalysis A: General, 486, 115-122(2014).
66. Muroyama, H., Tsuda, Y., Asakoshi, T., Masitah, H., Okanishi,
T., Matsui, T. and Eguchi, K., “Carbon Dioxide Methanation over
Ni Catalysts Supported on Various Metal Oxides,” Journal of
Catalysis, 343, 178-184(2016).
67. Li, Y., Men, Y., Liu, S., Wang, J., Wang, K., Tang, Y., An, W.,
Pan, X. and Li, L., “Remarkably Efficient and Stable Ni/Y2O3
Catalysts for CO2 Methanation: Effect of Citric Acid Addition,”
Applied Catalysis B: Environmental, 293, 120206(2021).
68. Cho, E. H., Park, Y.-K., Park, K. Y., Song, D., Koo, K. Y., Jung,
U., Yoon, W. R. and Ko, C. H., “Simultaneous Impregnation of Ni
and An Additive via One-step Melt-infiltration: Effect of Alkaline-
earth Metal (Ca, Mg, Sr, and Ba) Addition on Ni/γ-Al2O3
for CO2 Methanation,” Chemical Engineering Journal, 428, 131393
(2022).
69. Quindimil, A., Bacariza, M. C., González-Marcos, J. A., Henriques,
C. and González-Velasco, J. R., “Enhancing the CO2 Methanation
Activity of γ-Al2O3 Supported Mono-and Bi-metallic Catalysts
Prepared by Glycerol Assisted Impregnation,” Applied Catalysis
B: Environmental, 296, 120322(2021).
70. Dai, Y., Xu, M., Wang, Q., Huang, R., Jin, Y., Bian, B., Tumurbaatar,
C., Ishtsog, B., Bold, T. and Yang, Y., “Enhanced Activity
and Stability of Ni/La2O2CO3 Catalyst for CO2 Methanation by
Metal-carbonate Interaction,” Applied Catalysis B: Environmental,
277, 119271(2020).
71. Martínez, J., Hernández, E., Alfaro, S., López Medina, R., Valverde
Aguilar, G., Albiter, E. and Valenzuela, M. A., “High Selectivity
and Stability of Nickel Catalysts for CO2 Methanation: Support
Effects,” Catalysts, 9(1), 24(2018).
72. Quindimil, A., De-La-Torre, U., Pereda-Ayo, B., González-Marcos,
J. A. and González-Velasco, J. R., “Ni Catalysts with La as Promoter
Supported over Y-and BETA-zeolites for CO2 Methanation,”
Applied Catalysis B: Environmental, 238, 393-403(2018).
73. Westermann, A., Azambre, B., Bacariza, M., Graça, I., Ribeiro,
M., Lopes, J. and Henriques, C., “Insight into CO2 Methanation
Mechanism over NiUSY Zeolites: An Operando IR Study,” Applied
Catalysis B: Environmental, 174, 120-125(2015).
74. Huanling, S., Jian, Y., Jun, Z. and Lingjun, C., “Methanation of
Carbon Dioxide over a Highly Dispersed Ni/La2O3 Catalyst,”
Chinese Journal of Catalysis, 31(1), 21-23(2010).