Overall
- Language
- korean
- Conflict of Interest
- In relation to this article, we declare that there is no conflict of interest.
- Publication history
-
Received November 18, 2024
Revised February 10, 2025
Accepted February 13, 2025
Available online May 1, 2025
-
This is an Open-Access article distributed under the terms of the Creative Commons Attribution Non-Commercial License (http://creativecommons.org/licenses/bync/3.0) which permits unrestricted non-commercial use, distribution, and reproduction in any medium, provided the original work is properly cited.
Most Cited
CO2 흡수탑 내 최적 펌프어라운드 설치와 핀치 분석 기반 열통합을 이용한 전기화 블루수소 공정의 에너지 최적화
Energy Optimization of the Electrified Blue Hydrogen Process Using the Optimal Installation of Pumparound Flow in CO2 Absorber and Heat Integration Based on Pinch Analysis
https://doi.org/10.9713/kcer.2025.63.2.105106
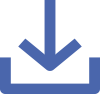
Abstract
온실가스를 배출하지 않고 수소를 생산할 수 있는 기술로서 최근 블루수소 제조기술이 주목받고 있으며, 천연가스 의 스팀개질반응과 수성가스전환반응을 이용하여 수소를 생산하되, 이 과정에서 생산되는 CO2는 별도의 포집공정을 통해 제거하는 것을 특징으로 한다. 하지만 스팀개질반응의 온도가 높고 흡열반응이므로 다량의 에너지를 공급해 주 어야 하며, CO2 포집 공정도 흡수제 재생에 많은 양의 에너지가 소모된다. 이에 본 연구에서는 전기에너지를 이용해 스팀개질반응에 필요한 열에너지를 공급한다는 가정 하에 흡수탑 내 펌프어라운드 흐름을 최적으로 배치하고 수소 생 산부와 CO2 포집부 간 최적의 열교환망을 구축하여 흡수제 재생에 필요한 에너지를 포함해 블루수소 제조공정 전체 에너지 소모량을 단계적으로 감소시키고자 하였다. 공정 시뮬레이션을 이용한 연구 결과, 흡수탑 내 펌프어라운드 유 입단수를 8단으로 지정할 경우, 블루수소 제조공정 전체의 가열유틸리티와 냉각유틸리티 소모량이 각각 9%, 10% 감 소하였으며, 핀치 분석 기반 최적 열통합을 적용한 경우 추가로 각각 29%, 42% 더 감소하는 결과를 보였다. 흡수제 재생탑의 재비기 에너지 소모량만 봤을 때는 총 51% 감소하는 효과를 보여 더 경제적인 방법으로 CO2 포집과 수소 생산이 가능할 것으로 예상된다.
Blue hydrogen manufacturing technology has recently attracted attention as a technology that can produce
hydrogen without emitting greenhouse gases. The characteristics of this technology are that it produces hydrogen by using the steam methane reforming and water-gas shift reactions of natural gas, and that the the CO2 produced in the process is removed by a separate capture process. However, since the temperature of the steam methane reforming reaction is high and the reaction is endothermic, a large amount of energy should be supplied, and the CO2 capture process also consumes a large amount of energy to regenerate the absorbent. In this study, under the assumption of electrified reformer, we aimed to significantly reduce the overall energy consumption of the blue hydrogen manufacturing process, including the energy required to regenerate the absorbent, by optimizing the location of a pumparound flow in the absorber and constructing the optimal heat exchange network. The results showed that the total heating and cooling utility consumption of this process was reduced by 9% and 10%, respectively, when the return stage of the pumparound flow in the absorber was set to eight, and further reduced by 29% and 42%, respectively, by performing heat integration. By implementing the two proposed methods, the energy consumption in the reboiler of the absorbent regenerator was also reduced by 51%, leading to the possibility of an economically feasible blue hydrogen process.
References
Institute of Gas, 27(3), 77-83(2023).
2. Ishaq, H., Dincer, I. and Crawford, C., “A Review on Hydrogen Production and Utilization : Chanllenges and Opportunities,” Hydrogen Energy, 47, 26238-26264(2022).
3. Do, T. N., Kwon, H. E., Park, M. S., Kim, C. S., Kim, Y. T. and Kim, J. Y., “Carbon-neutral Hydrogen Production from Natural Gas via Electrified Steam Reforming : Techno-economic-environmental Perspective,” Energy Conversioin and Management, 279, 116758 (2023).
4. Karimi, M., Hillestad, M. and Svendsen, H. F., “Investigation of Intercooling Effect in CO2 Capture Energy Consumption,” Energy Procedia, 4, 1601-1607(2011).
5. Mullen, D., Herraiz, L., Gibbins, J. and Lucquiaud, M., “On the Cost of Zero Carbon Hydrogen: A Techno-economic Analysis of Steam Methane Reforming with Carbon Capture and Storage,” Greenhouse Gas Control, 126, 103904(2023).
6. Pinto, D. D. D., Limpach, J. M. and Knuutila, H. K., “Simulationbased Assessment of the Potential of Offshore Blue Hydrogen
Production with High CO2 Capture Rates with Optimised Heat Recovery,” Gas Science and Engineering, 121, 205177(2024).
7. Limpach, J. M., Pinto, D. D. D. and Knuutila, H. K., “Heat Integration Study of CO2 Capture in Blue Hydrogen Production,” Chemical Engineering Transactions, 99, 373-378(2023).
8. Pedrozo, A., Valderrama-Ríos, C. M., Zamarripa, M. A., Morgan, J., Osorio-Su´arez, J. P., Uribe-Rodríguez, A., Diaz, M. S. and Biegler, L. T., “Optimization of CO2 Capture Plants with Surrogate Model Uncertainties,” Computers and Chemical Engineering, 186, 108709(2024).
9. Noh, H. J., Kang, K. G., Huh, C. and Lee, H. S., “Life Cycle Assessment of Carbon Capture and Storage in the Natural Gas Steam Methane Reforming Process for Hydrogen Production,” Energy &
Climate Change, 18(2), 179-193(2023).
10. Nozaeim, A. A., Tavasoli, A., Mortaheb, H. R. and Mafi, M., “CO2 Absorption/desorption in Aqueous DEEA/MDEA and Their Hybrid Solutions with Sulfolane,” Natural Gas Science and Engineering, 76, 103219(2020).
11. Jang, W. J., Yoon, Y. I., Park, S. D., Rhee, Y. W. and Baek, I. H., “Absorption of CO2 Using Mixed Aqueous Solution of N-methyldiethanolamine with Piperazine for Pre-comvustion CO2 Capture,”
Applied Chemistry for Engineering, 19(6), 645-651(2008).
12. Zhang, Y., Rochelle, G. T., “Absorber Performance with High CO2,” Energy Procedia, 63, 1329-1338(2014).
13. Tsay, C., Patiison, R. C., Zhang, Y., Rochelle, G. T. and Baldea, M., Rate-based Modeling and Economic Optimization of Nextgeneration Amince-based Carbon Capture Plants,” Aspentech Workshop Material, 252, 113379(2019).
14. Improved Energy Efficiency Through Heat Integration, Aspentech Workshop Material.
15. Wi, J. H., Choi, C. K., Joo, H. S., Cho, Y. S. and Kim, H. Y., “Heat Exchanger Network Synthesis by Using Exergy Analysis and Pinch Design Method,” Korean Institute of Chemical Engineers, 30(4), 406-414(1992).
16. Kemp, I. C., “Pinch Analysis and Process Integration: A User Guide on Process Integration for the Efficient Use of Energy,” 2nd ed., Butterworth-Heinemann, Oxford, UK(2007).