Articles & Issues
- Language
- korean
- Conflict of Interest
- In relation to this article, we declare that there is no conflict of interest.
- Publication history
-
Received June 18, 2024
Revised July 7, 2024
Accepted July 7, 2024
-
This is an Open-Access article distributed under the terms of the Creative Commons Attribution Non-Commercial License (http://creativecommons.org/licenses/bync/3.0) which permits unrestricted non-commercial use, distribution, and reproduction in any medium, provided the original work is properly cited.
Latest issues
젖산 산화효소-카탈라아제-미토콘드리아 전극 제작 및 특성 분석
Fabrication and Characterization of Lactate Oxidase-catalase-mitochondria Electrode
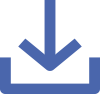
Abstract
젖산 전극은 환자의 건강상태와 스트레스 수준, 및 운동선수의 피로도를 실시간으로 모니터링하는 젖산 센서 또는
젖산 연료전지 전극으로 활용될 수 있다. 본 연구에서는 젖산 산화효소, 카탈라아제, 미토콘드리아로 구성된 고성능 전
극을 제작하고 전극의 표면분석 및 전기화학적 특성을 조사하였다. 단일벽 탄소나노튜브로 개질된 탄소종이(CPSWCNT)
는 개질 전보다 전기 전도성이 크게 향상되었다. 젖산 산화효소, 카탈라아제, 그리고 미토콘드리아가 부착된
전극(CP-SWCNT-LOx-Cat-Mito)은 젖산 산화효소와 카탈라아제가 부착된 전극에 비하여 많은 전류를 생산하였다. 빌
리루빈 산화효소(BOD)가 부착된 전극(CP-SWCNT-BOD)이 생산하는 환원전류량은 전해질의 산소 존재 유무에 따라
크게 영향을 받았다. CP-SWCNT-LOx-Cat-Mito (anode)와 CP-SWCNT-BOD (cathode)로 구성된 연료전지는 133 μA/
cm2로 방전 시 0.2 V의 셀 전위를 유지하며 29 μW/cm2의 전력을 생산하였다. 본 연구결과는 미토콘드리아가 젖산 센
서 및 연료전지 성능 향상에 필수적인 생체물질임을 시사한다.
The lactate electrode can be utilized either as an electrode for lactate sensor to monitor the patient's health
status, stress level, and athlete's fatigue in real time or lactate fuel cell. In this study, we fabricated a high-performance
electrode composed of lactate oxidase, catalase, and mitochondria, and investigated the surface analysis and electrochemical
properties of this electrode. Carbon paper modified with single-walled carbon nanotubes (CP-SWCNT) had significantly
improved electrical conductivity compared to before modification. The electrode to which lactate oxidase, catalase, and
mitochondria were attached (CP-SWCNT-LOx-Cat-Mito) produced a higher current than the electrode to which lactate
oxidase and catalase were attached. The amount of reduction current produced by the bilirubin oxidase (BOD)-attached
electrode (CP-SWCNT-BOD) was greatly affected by the presence or absence of oxygen in the electrolyte. The fuel cell
composed of CP-SWCNT-LOx-Cat-Mito (anode) and CP-SWCNT-BOD (cathode) produced maximum power (29 μW/
cm2) at a discharge current density of 133 μA/cm2. From this study, we had proved that mitochondria is essential for
improving lactate sensor and fuel cell performance.
References
on Electrochemical Lactate Detection: A Comprehensive Review,”
Biochem. Biophys. Rep., 5, 35-54(2016).
2. Madden, J., Vaughan, E., Thompson, M., Riordan, A. O., Galvin,
P., Lacopino, D. and Teixeira, S. R., “Electrochemical Sensor for
Enzymatic Lactate Detection Based on Laser-scribed Graphitic
Carbon Modified with Platinum, Chitosan and Lactate Oxidase,”
Talanta, 246, 123492(2022).
3. Derbyshire, P. J., Barr, H., Davis, F. and Higson, S. P., “Lactate
in Human Sweat: A Critical Review of Research to the Present
Day,” J. Physiol. Sci., 62, 429-440(2012).
4. Chung, M., Fortunato, G. and Radacsi, N., “Wearable Flexible
Sweat Sensors for Healthcare Monitoring; A Review,” J. R. Soc.
Interface, 16, 20190217(2019).
5. Shitanda, I., Takamatsu, K., Niiyama, A., Mikawa, T., Hoshi, Y.,
Itagaki, M. and Tsujimura, S., “High-power Lactate/O2 Enzymatic
Biofuel Cell Based on Carbon Cloth Electrodes Modified with
MgO-templated Carbon,” J. Power Sources, 436, 226844(2019).
6. Bandodkar, A. J., You, J.-M., Kim, N.-H., Gu, Y., Kumar, R.,
Mohan, A. M. V., Kurniawan, J., Imani, S., Nakagawa, T., Parish,
B., Parthasarathy, M., Mercier, P. P., Xu, S. and Wang, J., “Soft,
Stretchable, High Power Density Electronic Skin-based Biofuel
Cells for Scavenging Energy from Human Sweat,” Energy Environ.
Sci., 10, 1581-1589(2017).
7. Choi, H., Yeo, M., Kang, Y., Kim, H. J., Park, S. G., Jang, E.,
Park, S. H., Kim, E. and Kang, S., “Lactate Oxidase/Catalase-
Displaying Nanopaticles Efficiently Consume Lactate in The Tumor
Microenvironment to Effectively Suppress Tumor Growth,” J.
Nanobiotechnol., 21, 5(2023).
8. Andrus, L. P., Unruh, R., Wisniewski, N. A. and McShane, M.
J., “Characterization of Lactate Sensors Based on Lactate Oxidase
and Palladium Benzoporphyrin Immobilized in Hydrogels,” Biosensors,
5, 398-416(2015).
9. Sokic-Lazic, D., Andrade, A. R. D. and Minteer, S. D., “Utilization
of Enzyme Cascade for Complete Oxidation of Lactate in an
Enzymatic Biofuel Cell,” Electrochim. Acta, 56, 10772-10775
(2011).
10. Shi, K., Selvarajan, V., Yang, Y.-Y. and Kim, C.-J., “Fabrication
and Characterization of Carbon Nanotube-modified Carbon Paperbased
Lactate Oxidase-catalase Electrode,” Korean Chem. Eng.
Res., 61, 1-8(2023).
11. McKee, T. and Mckee J. R., Biochemistry: The Molecular Basis
of Life, 5th ed., Oxford, New York, NY (2013).
12. Pichardo, S., Gutierrez-Praena, D., Puerto, M., Sanchez, E., Grilo,
A., Camean, A. M. and Jos, A., “Oxidative Stress Response to
Carboxylic Acid Functionalized Single Wall Carbon Nanotubes
on the Human Intestinal Cell Line Caco-2,” Toxicol In Vitro, 26,
672-677(2012).
13. Timur, S., Haghighi, B., Tkac, J., Pazarlioglu, N., Telefoncu, A.,
and Gorton, L., “Electrical Wiring of Pseudomonas putida and
Pseudomonas fluorescens with Osmium Redox Polymers,” Bioelectrochemistry,
71, 38-45(2007).
14. Kim, H.-H., Mano, N., Zhang, Y., and Heller, A., “A Miniature
Membrane-less Biofuel Cell Operating under Physiological Condition
at 0.5 V,” J. Electrochem. Soc., 150, A209-A213(2003).
15. Zaib, Q. and Ahmad, F., “Optimization of Carbon Nanotube
Dispersions in Water Using Response Surface Methodology,”
ACS Omega, 4, 86-92(2019).
16. Koh, B. and Cheng, W., “The Impact of Sonication on the Surface
Quality of Single-Walled Carbon Nanotubes,” Pharm. Nanotechnol.,
104, 2594-2599(2015).
17. Neikirk, K., Marshall, A. G., Kula, B., Smith, N., Leblanc, S.,
and Hinton Jr., A., “MitoTracker: A Useful Tool in Need of Better
Alternatives,” Eur. J. Cell Biol., 102, 151371(2023).
18. Baracca, A., Sgarbi, G., Solaini, G., and Lenaz, G., “Rhodamine
123 As a Probe of Mitochondrial Membrane Potential: Evaluation
of Proton Flux Through F0 during ATP Synthesis,” Biochim. Biophys.
Acta, 1606, 137-146(2003).
19. Manke, et al., “Effect of Fiber Length on Carbon Nanotube-
Induced Fibrogenesis,” Int. J. Mol. Sci., 15, 7444-7461(2014).
20. Zhang, Y, Selvarajan, V., Shi, K. and Kim, C.-J., “Fabrication
and Characterization of Glucose-Oxidation-Trehalase Electrode
Based on Nanomaterial-Coated Carbon Paper,” RSC Adv., 13,
33918-33928(2023).
21. Cai, B., Li, M., Zhou, J. Tan, L., Li, D. and Ao, Z., “Effect of
Oxygen-Containing Functional Groups at SWCNT on the Formation
of Sodium and Lithium Dendrites,” Surf. Interfaces., 40,
103074(2023).
22. Khan, N., Anwer, A. H., Ahmad, A., Sabir, S., Sevda, S. and
Khan, M. Z., “Investigation of CNT/PPy-Modified Carbon Paper
Electrodes Under Anaerobic and Aerobic Conditions for Phenol
Bioremediation in Microbial Fuel Cells,” ACS Omega., 5, 471-
480(2020).
23. Kuznetsova, A. et al., “Oxygen-containing Functional Groups
on Single-wall Carbon Nanotubes: NEXAFS and Vibrational
Spectroscopic Studies,” J. Am. Chem. Soc., 123, 10699-10704(2001).
24. Benko, Aleksandra. et al., “Covalently Bonded Surfaces Functional
Groups on Carbon Nanotubes: From Molecular Modeling
to Practical Applications,” Nanoscale, 13, 10152-10166(2001).
25. Barreca, D., Neri, G., Scala, A., Fazio, E., Gentile, D., Rescifina,
A. and Piperno, A., “Covalently Immobilized Catalase on Functionalized
Graphene: Effect on the Activity, Immobilization Efficiency,
and Tetramer Stability,” Biomater. Sci., 6, 3231-3240(2018).
26. Willey, J. M., Sherwood, L. M. and Woolverton, C. J., Prescott,
Harley, and Klein’s Microbiology, 7th ed., McGraw-Hill, New
York, NY(2008).
27. Jayakumar, K., Bennett, R. and Leech, D., “Electrochemical Glucose
Biosensor Based on an Osmium Redox Polymer and Glucose
Oxidase Grafted to Carbon Nanotubes: A Design-of-Experiments
Optimisation of Current Density and Stability,” Electrochim. Acta.,
371, 137845(2021).
28. Vasylieva, N., Barnych, B., Meiler, A., Maucler, C., Pollegioni,
L., Lin, J.-S., Barbier, D. and Marinesco, S., “Covalent Enzyme
Immobilization by Poly(Ethylene Glycol) Diglycidyl Ether (PEGDE)
for Microelectrode Biosensor Preparation,” Biosens. Bioelectron.,
26, 3993-4000(2011).
29. Alhansa, R., Singhb, A., Singhala, C., Naranga, J., Wadhwaa, S.
and Mathurb, A., “Comparative Analysis of Single-walled and
Multi-walled Carbon Nanotubes for Electrochemical Sensing of
Glucose on Gold Printed Circuit Boards,” Mater. Sci. Eng., C90,
273-279(2018).
30. Yuwen, et al., “Carbon Nanotubes: A Powerful Bridge for Conductivity
and Flexibility in Electrochemical Glucose Sensors,” J.
Nanotechnol., 21, 320(2023).
31. Sokic-Lazic, D., Andrade, A. R. and Minteer, S. D., “Utilization
of Enzyme Cascades for Complete Oxidation of Lactate in an
Enzymatic Biofuel Cell,” Electrochim. Acta., 56, 10772-10775(2011).
32. Mannella, C. A. and Wang, Q., “Permeability of the Mitochondrial
Outer Membrane to Organic Cations,” Biochim. Biophys. Acta.,
981, 363-366(1989).
33. Nishikawa, M., Nojima, S., Akiyama, T., Sankawa, U. and Inoue,
K., “Interation of Digitonin and Its Analogs with Membrane
Cholesterol,” J. Biochem., 96, 1231-1239(1984).
34. Shitanda, I., Hirano, Kai, Loew, N., Watanabe, H., Itagaki, M.
and Mikawa, T., “High-performance, Two-step/Bi-enzyme Lactate
Biofuel Cell with Lactate Oxidase and Pyruvate Oxidase,”
J. Power Sources, 498, 229935(2021).